Fundamentals of momentum heat and mass transfer solutions – Fundamentals of Momentum, Heat, and Mass Transfer Solutions unveils the intricate interplay of these fundamental principles, providing a comprehensive guide to their practical applications and theoretical underpinnings. This narrative delves into the governing equations, analytical and numerical methods, and real-world applications that shape our understanding of fluid dynamics and transport phenomena.
From the basic concepts of momentum, heat, and mass transfer to advanced topics like turbulence and multiphase flow, this guide equips readers with a thorough understanding of these essential principles and their impact on engineering and scientific disciplines.
1. Basic Concepts of Momentum, Heat, and Mass Transfer
Momentum, heat, and mass transfer are fundamental processes that occur in nature and engineering systems. These processes involve the transfer of momentum, heat, and mass between different regions of a fluid or between a fluid and a solid surface.
The basic principles of momentum, heat, and mass transfer can be explained using the conservation laws of mass, momentum, and energy. These laws state that the total mass, momentum, and energy of a system remain constant over time, except for any mass, momentum, or energy that is transferred in or out of the system.
Momentum transfer is the process by which momentum is transferred between different regions of a fluid or between a fluid and a solid surface. Momentum transfer occurs due to the interaction of molecules or particles in the fluid. The rate of momentum transfer is proportional to the velocity gradient in the fluid.
Heat transfer is the process by which heat is transferred between different regions of a fluid or between a fluid and a solid surface. Heat transfer occurs due to the random motion of molecules or particles in the fluid. The rate of heat transfer is proportional to the temperature gradient in the fluid.
Mass transfer is the process by which mass is transferred between different regions of a fluid or between a fluid and a solid surface. Mass transfer occurs due to the diffusion of molecules or particles in the fluid. The rate of mass transfer is proportional to the concentration gradient in the fluid.
Examples of Real-World Applications of Momentum, Heat, and Mass Transfer Principles
- The design of aircraft wings to generate lift and control flight.
- The design of heat exchangers to transfer heat between two fluids.
- The design of chemical reactors to control the rate of chemical reactions.
- The design of medical devices to deliver drugs or heat to specific regions of the body.
Different Types of Fluids and Their Properties, Fundamentals of momentum heat and mass transfer solutions
Fluids can be classified into two main types: Newtonian fluids and non-Newtonian fluids.
Newtonian fluids are fluids that have a constant viscosity, which means that their resistance to flow is directly proportional to the velocity gradient. Examples of Newtonian fluids include water, oil, and air.
Non-Newtonian fluids are fluids that do not have a constant viscosity. Their resistance to flow can vary depending on the velocity gradient, temperature, or other factors. Examples of non-Newtonian fluids include ketchup, toothpaste, and blood.
2. Governing Equations and Constitutive Relations: Fundamentals Of Momentum Heat And Mass Transfer Solutions
The governing equations for momentum, heat, and mass transfer are derived from the conservation laws of mass, momentum, and energy. These equations are partial differential equations that describe the conservation of mass, momentum, and energy in a fluid.
Physical Meaning of Each Term in the Governing Equations
The governing equations for momentum, heat, and mass transfer contain several terms that represent different physical phenomena. These terms include:
- The velocity gradient, which represents the rate of change of velocity with respect to position.
- The pressure gradient, which represents the rate of change of pressure with respect to position.
- The temperature gradient, which represents the rate of change of temperature with respect to position.
- The concentration gradient, which represents the rate of change of concentration with respect to position.
- The viscosity, which represents the resistance of a fluid to flow.
- The thermal conductivity, which represents the ability of a fluid to conduct heat.
- The mass diffusivity, which represents the ability of a fluid to diffuse mass.
Constitutive Relations Used to Model Fluid Behavior
Constitutive relations are equations that relate the stress tensor to the velocity gradient in a fluid. These relations are used to model the behavior of fluids under different conditions.
The most common constitutive relation is the Newtonian constitutive relation, which states that the stress tensor is proportional to the velocity gradient. This relation is valid for Newtonian fluids.
Non-Newtonian fluids require more complex constitutive relations to model their behavior. These relations can be quite complex and may depend on the velocity gradient, temperature, or other factors.
User Queries
What are the key applications of momentum, heat, and mass transfer principles?
These principles find widespread applications in various fields, including aerospace engineering, chemical engineering, environmental engineering, and biomedical engineering, to optimize system design, predict fluid behavior, and enhance energy efficiency.
How do analytical and numerical methods differ in solving momentum, heat, and mass transfer problems?
Analytical methods provide exact solutions for simplified problems, while numerical methods, such as finite element analysis and computational fluid dynamics (CFD), offer approximate solutions for complex geometries and boundary conditions.
What are the challenges encountered in advanced topics like turbulence and multiphase flow?
Turbulence involves complex flow patterns and requires advanced modeling techniques, while multiphase flow deals with the interaction of multiple fluids, posing challenges in predicting their behavior and optimizing system performance.
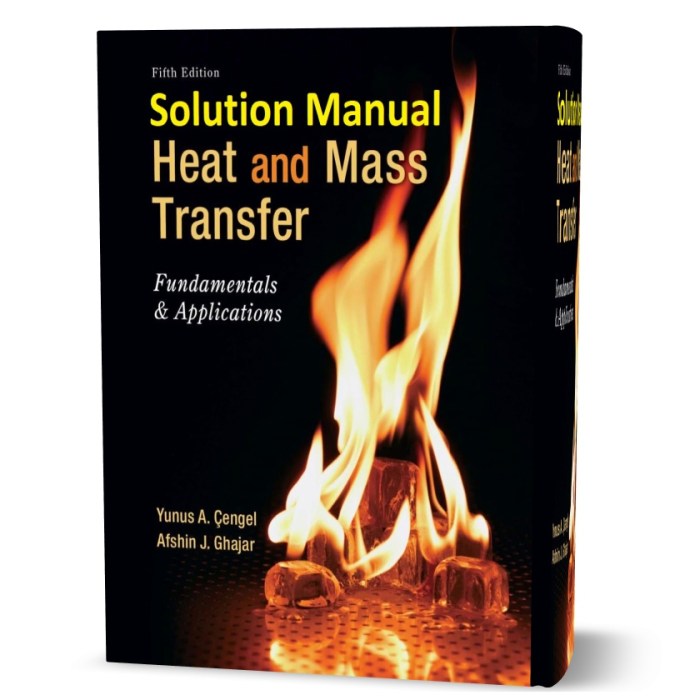